Voltage Rating
How to select the voltage rating of a lightning arrester?
For that, we have to consider two conditions: one is the effectively earthed condition, and the other is the non-effectively earthed condition.
An effectively earthed condition means a star-connected system where the star point is solidly earthed, like in our 132 KV system, 220 KV system, or 400 KV system. Here, the three phases RYB are star-connected in the transformer, and the star point of the transformer is earthed directly or solidly. But in the 33 KV system, in many power utilities, a delta-connected transformer winding is used. In a delta-connected winding, there is no provision for direct earthing because, in a delta connection, there is no star point or common point for the three-phase winding. This is called a non-effectively earthed system.
Now, if we consider a star-connected system and imagine there is a fault in one phase, since the star point is solidly earthed, it is not possible for the other phases to increase the voltage beyond 80% of phase to phase voltage or simply 80% of line voltage of the system.
But in a delta connection, if any fault occurs in any of the phases, the voltage of the other two phases may reach up to √3 times of their phase voltage or little bit higher. In an effectively earthed system, during a fault in any of the phases, the voltage of the other two phases will not exceed the healthy phase voltage. But in a non-effectively earthed system, the voltage of the healthy phases goes up to 100% or even little bit more of the line voltage. The rated voltage of a lightning arrester for an effectively earthed system is generally 0.8 times the phase to phase voltage or rated line voltage of the system. For non-effectively earthed system the voltage rating of a lighting arrester is 100% or even more of the system line voltage.
Say, for a 132 KV system, the rated voltage of the selected lightning arrester will be 0.8 times of 145 KV, because the highest system voltage of 132 KV is 145 KV, or 1.1 times 132 KV. Considering 132 KV, we have to write 0.8 × 1.1 × 132 KV, and this is the same as 0.8 × 145 KV, which is around 120 KV.
Let us again take an example of a 220 KV system. The 220 KV system is also solidly earthed, and here, the rated voltage of the lightning arrester will be 0.8 × 245 KV, which is nearly equal to 198 KV, because 220 KV has the highest system voltage of 245 KV. This is again 1.1 times, or 110% of 220 KV. So, 198 KV is the rated voltage of the selected lightning arrester for a 220 KV system.
Now come to the 33 KV system. The 33 KV system, as I mentioned, is normally non-effectively earthed. So, in the 33 KV system, the selected lightning arrester will have a rated voltage of 33 KV × 1.1, or 36 KV only. But there may be some safety factor multiplied, and that will ultimately be chosen as 42 KV for most utilities. Choosing 42 KV instead of 36 KV is simply providing some percentage of margin. But if you go for a direct 36 KV, that can also be chosen.
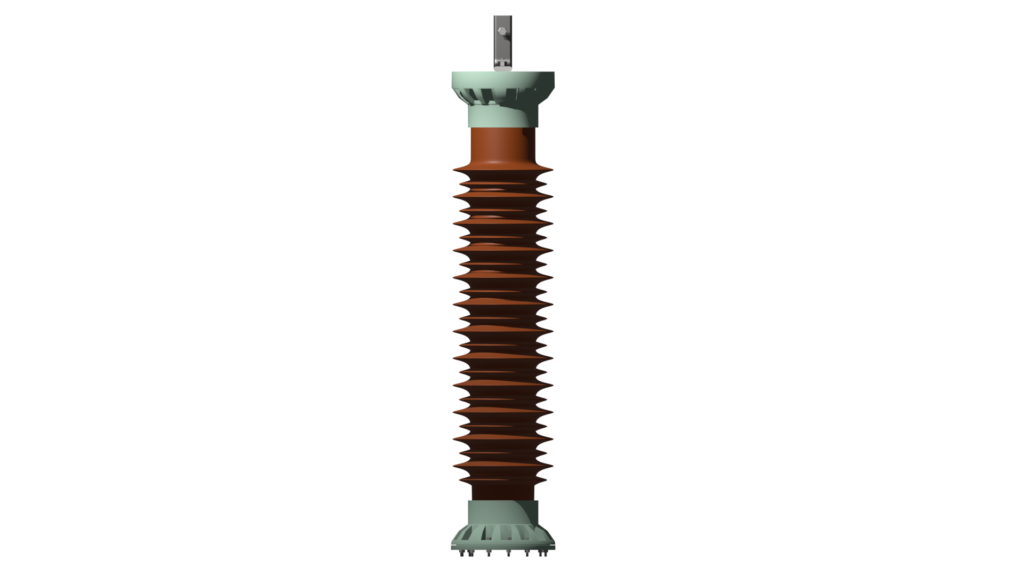
What is the Maximum Continuous Operating Voltage (MCOV) of a lightning arrester?
A lightning arrester is normally connected between the phase and earth. There are two voltage conditions between phase and earth: one occurs during an earth fault in other phases, and the other under normal (healthy) conditions.
When there is an earth fault in other phases, the voltage of the phase where the lightning arrester is connected will rise. At that time, the lightning arrester should not conduct any current, because it is not supposed to operate during earth fault conditions. It is designed to operate only during lightning or switching impulses.
The lightning arrester must withstand this high voltage temporarily, but since this voltage is not continuous, it is quickly cleared by the protection system. According to Indian standards, this voltage rating refers to the power frequency voltage that the arrester can withstand for a limited duration (typically 10 seconds) without conducting any current to earth, except for its nominal leakage current.
Under normal system conditions (i.e., healthy condition), the lightning arrester is simply exposed to the phase voltage of the system, which is the line-to-earth voltage. Even in this state, it should not conduct any current to earth except its nominal leakage current.
For this reason, another voltage rating is assigned to a lightning arrester: the Maximum Continuous Operating Voltage (MCOV). The earlier discussed voltage (withstand rating for 10 seconds) is temporary, whereas MCOV refers to the continuous voltage that appears across the arrester when it is connected between phase and earth. This is the phase voltage, i.e., line voltage divided by √3.
This voltage is applied continuously, and the arrester must not conduct any current other than allowable leakage. To ensure a safety margin, the MCOV of a lightning arrester must be slightly higher than the system’s phase voltage.
For example, in a 132 kV system, the phase voltage is 132 / √3 ≈ 76.2 kV. After adding the necessary safety margin, the MCOV becomes 102 kV. However, the rated voltage of the lightning arrester for such a system is typically 120 kV.
So, a lightning arrester has two main voltage ratings:
- Rated Voltage – For example, 120 kV, which it can withstand for 10 seconds during fault conditions.
- Maximum Continuous Operating Voltage (MCOV) – For example, 102 kV, which it must withstand continuously under normal operating conditions.
Nominal Discharge Current
Depending on this current rating, lightning arresters are classified into different categories: distribution class and station class lightning arresters.
In a distribution class lightning arrester, the rated nominal discharge current is taken as 5 KA or below. For station class lightning arresters, the rated nominal discharge current is taken as 10 KA for 132 kV systems and 10 to 20 KA for 220 kV and above systems. The nominal discharge current plays a significant role during choosing of a lightning arrester. It is defined as the current that will discharge through the arrester during a surge, and the waveform of this current is specified as an 8/20 microseconds impulse waveform.
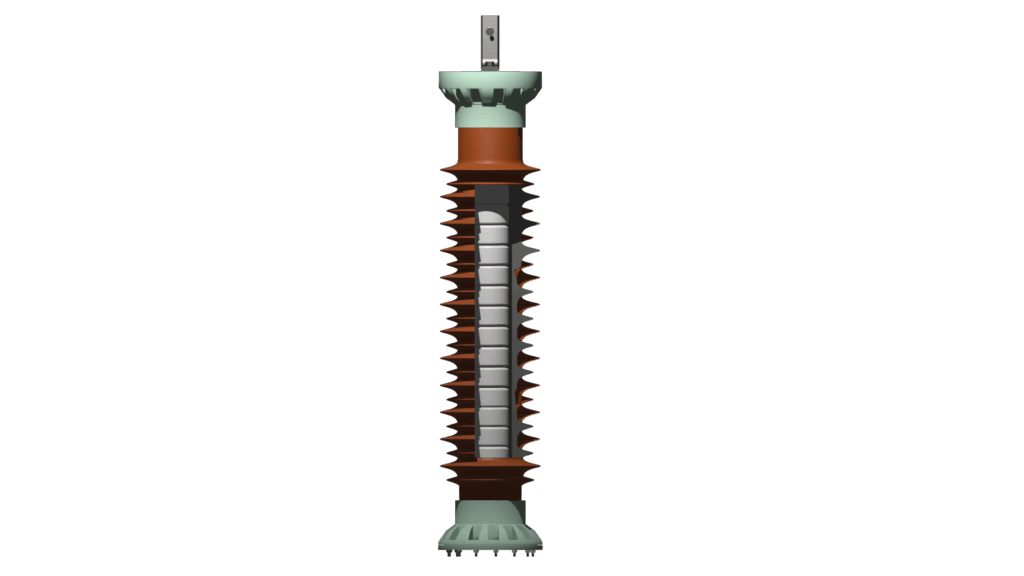
Residual Voltage of a Lightning Arrester
When the discharge current passes through the arrester, due to the inherent impedance of the arrester, a voltage develops across it. This voltage is called the residual voltage. It must be less than the Basic Insulation Level (BIL) of the equipment connected to the system where the lightning arrester is installed for protection. The lightning impulse withstand capability of an equipment is rated with the peak impulse voltage with a 1.2/50 microsecond waveform.
For example, suppose the transformer bushing connected to a 132 kV system has a lightning impulse withstand capability of 550KV. For a station class lightning arrester with a discharge current of 10 KA. For this current, the residual voltage developed across the arrester should be less than 550 kV peak. Otherwise, the arrester will fail to protect the equipment during actual lightning events.
Protective Ratio of a Lightning Arrester
Suppose for a 10 KA nominal discharge current, the residual voltage appearing across the arrester is 340 kilovolts. The protective ratio is defined as: \[ \text{Protective Ratio} = \frac{\text{Lightning Impulse Withstand Voltage of Equipment}}{\text{Residual Voltage of Arrester}} \]This is an important parameter used to choose the lightning arrester. Hence for the above mention transformer bushing the Protective Ration will be, \[ \text{Protective Ratio} = \frac{550}{340}=1.61 \]
Maximum Discharge Current
Another important current rating associated with a lightning arrester is the maximum discharge current rating, also called the high current impulse withstand capability. It is measured in kiloamperes peak and it follows a 4/10 microsecond waveform.
For a station class lightning arrester, the standard value of this current is 100 KA, and for a distribution class arrester, it is 65 KA as per Indian standards.
Very high discharge currents may occur, especially when a lightning stroke hits in close proximity to the arrester. However, the energy discharged during such high current events is not very significant due to the very short duration of the impulse — just 4/10 microseconds.
Energy Absorption Capability of a Lightning Arrester
When the rated discharge current flows through the body of the lightning arrester, a residual voltage is developed across it. If we multiply this discharge current I by the residual voltage E, we obtain the power (IE) dissipated across the lightning arrester during the discharge of the surge current (I).
If we then multiply that power by the duration for which the discharge current flows through the arrester, we get the energy. This energy is ultimately converted into heat, and the arrester’s body or structure must be capable of absorbing this energy without any permanent distortion or alteration of its characteristics.
This defines the energy absorption capability rating of a lightning arrester, which is expressed in kilojoules per kilovolt (kJ/kV).