As the name suggests, ferroresonance is a resonance phenomenon related to iron. Ferro means ‘iron’. Before understanding the ferroresonance, let us recall the concept of resonance first. Suppose, we have a series RLC circuit, across which a voltage source is connected. Say a current I flowing through the circuit.
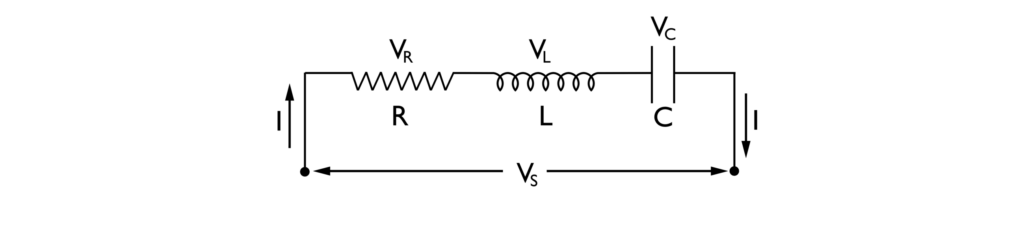
VL is the voltage developed across the inductor,
VC is the voltage developed across the capacitor,
VR is the voltage dropped across the resistor,
VS is the source voltage.
Therefore, we can write,\[ V_L=j2\pi fLI \;\;\&\;\; V_C=\frac{- jI}{2\pi fC} \]
If we draw the phasor diagram, we will see that VL is opposing VC. This is because VL lags the resistive voltage VR by 90°, and VC leads VR by 90°.
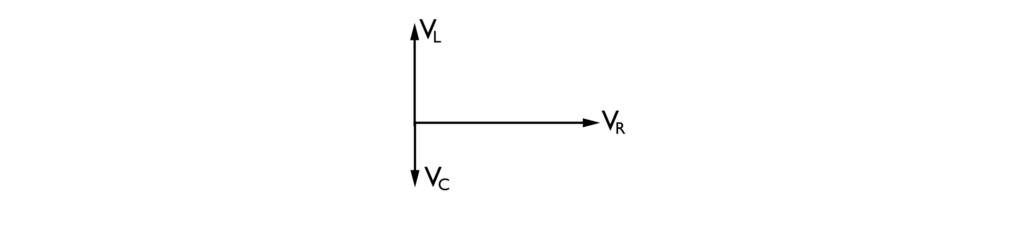
Now, if we change the frequency of the source voltage, the voltages across the inductor and capacitor will also change. This is because the inductive voltage is directly proportional to the source frequency, while the capacitive voltage is inversely proportional to it, as we can see from the above equations. Therefore, at a certain frequency, these two voltages will be equal in magnitude. Since the directions of these voltages are exactly opposite to each other, they will cancel each other out at that frequency.
Thus, at this frequency, the net opposing voltage to the source voltage becomes zero in the circuit. As a result, the current in the circuit reaches to a very high value. This frequency is known as the resonant frequency of that RLC circuit. The phenomenon is called resonance. Alternatively, we can say that at the resonant frequency, the RLC circuit behaves as a purely resistive circuit.
Now one question may arise in our mind that, as the frequency of our power system is constant, it is either 50 Hz or 60 Hz (America), how resonance can occur in the power system.
Suppose there is a 3-phase system that is not effectively earthed. In such a system, during a phase-to-earth fault, the voltage of the faulted phase drops close to zero, while the voltages of the other two phases may rise up to √3 times the normal phase-to-ground voltage. Due to this high voltage, the core of the potential transformer (PT) connected to those two phases may become saturated. As a result of this saturation, the inductance of the PT coil may vary. In other words, the inductance of a PT can be considered a non-linear inductance.
Again, when there is a fault in a phase, the voltage of that phase decreases, but at the same time the current through the PT coil increases rapidly. This increased current can also cause saturation of the PT.
Due to change in inductance, resonance occurs even at rated power frequency. During resonance, there will be an oscillation in the signal. If resistance of the circuit is quite low, the time constant of the circuit will be large. Therefore, this oscillation will not be damped soon and oscillation will continue for a prolong time.
Four Causes of Ferroresonance
Therefore, we can conclude that, there will be total four reasons due to which ferroresonance may occur:
- Non-linear inductance in the circuit
Due to PT core saturation, inductance of the PT circuit becomes nonlinear. - Presence of capacitance in the circuit
Due to the inherent capacitance of the circuit and system, there will always be a capacitance imposed in the PT circuit. - Presence of voltage source across the circuit
A PT is connected across the power circuit, which is nothing but the voltage source of the PT circuit. - Minimum resistance connected to the circuit
The relay and metering circuits basically have very low resistance. The resistance of the relays, meters and connecting leads are kept below a certain low limit. If these resistances increase, the burden will also increase and it’ll go beyond the specified limit. Beyond this limit of burden the PT operates beyond limit of errors which is not desirable.
If we remove any one of the four reasons, ferroresonance will not happen. But we cannot remove the non-linearity of the PT circuit. This is because the PT core has natural saturation. We also cannot remove the system’s capacitance. This is because many cables are used in the system today.
Now, as we have already discussed, saturation of the PT core occurs when the magnetic flux in the core exceeds its capacity. When a high current flows through the PT coil, the magnetic flux also increases and can cause the core to become saturated. So, if we can somehow control this extra flux, even when excess current is present, then ferroresonance can be avoided.
How to Minimize Ferroresonance in Potential Transformer
To achieve this, we can use a delta-connected circuit across all three PT secondaries, as shown. Under normal balanced condition, the vector sum of PT secondary voltages is zero. Therefore, no current flows through the delta secondary circuit. However, during a fault in any of the phases, this balance is disturbed. The vector sum is no longer zero, and a current begins to flow in the closed delta circuit.
This current produces a counter flux in the PT core. This counter flux limits the total flux in the core, preventing it from reaching saturation. As a result, the PT core remains unsaturated, and ferroresonance is avoided in the PT secondary.

Sometimes, a damping resistor is connected in the delta secondary circuit. During normal conditions, since no current flows in the delta, the resistor has no effect. Therefore, this additional resistor does not affect the PT burden. During a fault, this resistance comes into picture and acts as the damping resistance in the resonant circuit.